Rise Above the Grid!
- Dr Imtiaz Madni
- Sep 29, 2021
- 5 min read
This is the Microgrids Moment
A microgrid (MG) is a local energy grid with the control capability to operate separately from a conventional grid. It is emerging as a new energy solution thanks to the declining cost of energy storage and the increasing integration of renewable energy as technology improves.
Microgrids can be found in cities, neighbourhoods, mining operations and remote locations - Check out the ones in New York City and Brooklyn, Australia’s largest hybrid renewable energy microgrid, as well as the ones in remote locations like the Alcatraz and villages in Myanmar.
Navigant Research forecasted a value of US $31 billion in the global market for microgrids by 2027. Such growth is no surprise when you consider the issues that microgrids solve. For example, the Australian government has allocated a total fund of $25.6 (US $18.8) million to support twenty micro-grid projects in rural farming communities and remote areas, including communities that destructive bushfires had hit. Many of the companies involved in these projects are providing solutions where low-cost, low-maintenance renewable energy plays a key role in facilitating local services and improving resilience for affected communities in regional Australia while cutting emissions.

Figure 1. An example of a microgrid
Source: Reproduced from Chang (2018)
The Greener Grid
Microgrids have significant advantages of efficiency and fast power system restoration. Microgrids have benefits such as facilitating various distributed generation (DG) sources, allowing high penetration of renewable energy sources such as solar, wind or hydro. Moreover, microgrids provide local voltage and frequency regulation support and improve the reliability and power capacity of the grid.
In high-income economies, microgrids are capable of ensuring efficient, low-cost, reliable and clean high-quality energy needed to host facilities to stay competitive. For developing economies, microgrids can help remote rural communities get access to basic electrical necessities and improve their quality of life.
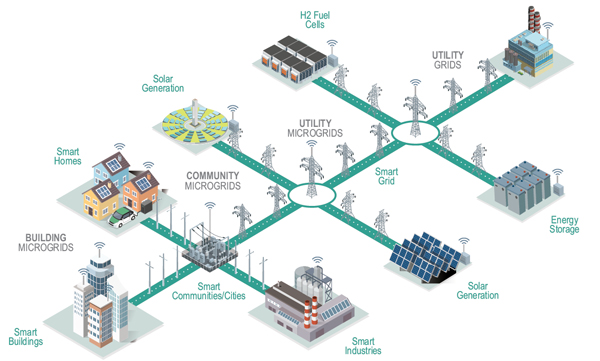
Figure 2: An example of distributed generation in smart cities
Source: Reproduced from Energy-Producing Retail Realty (2019)
Microgrids Control Methodologies
Control strategies and issues closely related to microgrid operation have been explored in several studies. A widely accepted method among the control strategies is based on a technique known as “droop control”. Other methods include a central controller with a hierarchical control scheme to optimise the operation of the microgrid during interconnected operation and an advanced sliding mode controller based on stability function to design suitable mathematical modelling with uncertain parameters to enhance the dynamics characteristics of a microgrid. Microgrid technologies can be classified into two modes of operation: grid-connected mode or islanded mode.
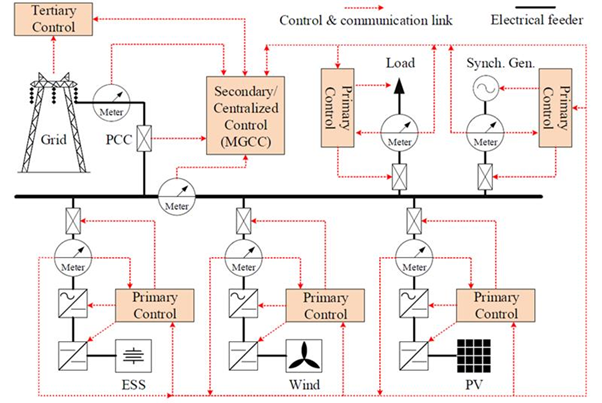
Figure 3: Example of a central controller for a typical microgrid
Source: Reproduced from Institute of Electrical and Electronics Engineers (IEEE) (2019)
Using a microgrid control system has two advantages:
The stability of microgrid operation in frequency and voltage is maintained, especially in the load changes or interconnection with other networks.
The active and reactive powers of the converter are regulated.
This article focuses on some of the most effective techniques for microgrid controls as follows:
P-Q (Active-Reactive Power) Control
Droop control
Voltage/frequency control
Current control methods
P-Q (Active-Reactive Power) Control
In microgrid systems, a public control, ie. PQ control strategy is used. PQ controls the voltage output of the inverter by injecting the active and reactive powers. This control is used in cases wherein the microgrid is not required or unable to provide voltage or frequency support as a current-controlled voltage source. With PQ control, the microgrid controller does not change the output settings of its microgrid in response to fluctuations in terminal voltage or frequency, which requires a reference frequency signal to operate.
During grid-connected operation, this reference frequency is supplied by the main grid. Meanwhile, this reference frequency must be supplied by another microgrid that operates on droop control in islanded operation.
Droop Control
A droop control method is typically used for controlling microgrids in islanded operations such as the conventional generators of power plants. In the islanded operation of converter-based DG (Distributed Generation) systems, this method can be adopted to share total load and control voltage magnitude and frequency in a special range. In this control unit, the active power of the total load can be shared among sources by drooping the frequency as a function of the active output power of the converter. The applicability of droop control is readily apparent by considering the relationships that dictate the power transfer in a two-inverter system.
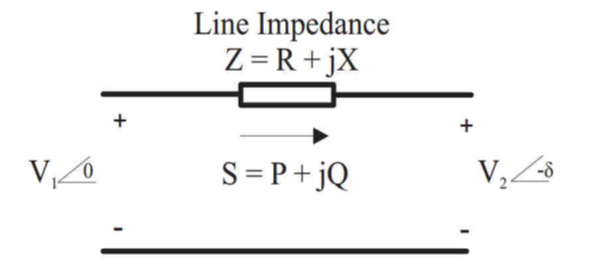
Figure 4: Droop control’s two-inverter system
Voltage/Frequency Control
The microgrid voltage control ensures that the voltage remains within the setpoint values by adjusting generated or consumed reactive power. A voltage controller is designed with the terminal voltages as input and compared with the reference voltage. The error voltage is filtered using a low pass filter and multiplied by a gain constant to obtain droop control of the voltage source converter. The output of the voltage regulation control block gives the reactive power that needs to be injected to maintain the terminal voltage according to the droop set value. Hence, the traditional frequency droop control through active power and voltage droop control through reactive power may not function very well on a low-voltage network-based microgrid. Thus, the voltage control should be implemented through active power and frequency control through reactive power generated in an inverter.
Current-mode Control (CMC)
Current-mode Control (CMC) is typically used to track the set point derived from droop or other control approaches. Notably, in this control approach, both loops have finite gain. The inner (current) loop acts as a disturbance that introduces a voltage and phase offset proportional to the load current. Therefore, changes in the load impedance would change the system transfer function, thereby changing the amplitude and phase at a given frequency.
Conclusion
There are more options for energy distribution via renewable energy such as solar and wind due to the recent technological advancement in microgrids. With weather events such as bushfires and floods becoming more frequent and intense, there is a challenge to extend power to affected areas during and after these events, highlighting the need for local energy solutions like microgrids. With this, the distribution network may become more complex and require good control strategies to manage its power while optimising renewable energy sources.
As a member of the Australian Microgrid Centre of Excellence (AMCOE), Divergent Engineering is moving towards the direction of renewable energy integration. Our team has the knowledge and expertise to integrate large-scale solar farms, battery energy storage systems and microgrid design and control systems for distributed energy resources. Contact us today to learn how we can help you!
About the author:
Dr Imtiaz Madni
Senior Electrical Engineer
Imtiaz comes from a solid technical background with diverse working experience in the energy industry as a senior electrical engineer and electrical project manager. He has a demonstrated history of working in the manufacturing, electrical systems, renewables, and higher education industries.
Comments